Revolutionizing Network Security with Quantum Cryptography
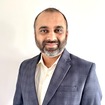
Introduction
In the ever-evolving landscape of cybersecurity, the advent of Quantum Cryptography stands as a groundbreaking paradigm shift, promising to revolutionize network security in ways previously thought impossible. Unlike traditional cryptographic methods, Quantum Cryptography harnesses the principles of quantum mechanics to create an unbreakable shield against malicious attacks. The quantum revolution is not just a theoretical concept; it is a tangible force reshaping the landscape of network security, paving the way for a new era of unparalleled protection in the interconnected world we navigate today. One real-world scenario where data security is crucial is in the banking sector. Banks and financial institutions deal with sensitive customer information on a daily basis, including personal and financial data such as account numbers, social security numbers, and transaction histories. Protecting this data from unauthorized access, theft, or misuse is paramount to maintaining trust and credibility with customers and regulatory compliance.
Modern encryption uses digital keys and certificates to ensure only the intended recipient can decode the information. Collectively, this is known as public key infrastructure (PKI).- Today, PKI is the trust fabric of the digital world, allowing us to authenticate connections, encrypt data as it’s shared across networks, and digitally sign software and documents. These core elements of security could be undermined if the mathematical algorithms used to encrypt and decrypt data could be cracked by a powerful computer. Today’s computers use binary “bits” composed of ones and zeros. Quantum computers process information using qubits, which aren’t ones or zeros, but the physical movements and positions of photons and electrons.
Here is an illustration of how Quantum encryption works:
Imagine there are two people, Alice and Bob, who want to send secure information to each other that no one else can intercept. With Quantum Key Distribution (QKD), Alice sends Bob a series of polarized photons over a fiber optic cable. This cable doesn’t need to be secured because the photons have a randomized quantum state.
If an eavesdropper, named Eve, tries to listen in on the conversation, she must read each photon to read the secret. Then she must pass that photon on to Bob. By reading the photon, Eve alters the photon’s quantum state, which introduces errors into the quantum key. This alerts Alice and Bob that someone is listening, and the key has been compromised, so they discard the key. Alice must send Bob a new key that isn’t compromised, and then Bob can use that key to read the secret. Refer to the illustration given below.
Note: This information was sourced from Quantum Xchange who was focused on building and selling the first quantum network in the U.S.
Challenges with Traditional Cryptography:
On 23rd October 2019, Google published a groundbreaking scientific research article announcing one of the “holy grails” of quantum computing research: For the first time ever, a quantum computer had solved a mathematical problem faster than the world's fastest supercomputer.
The security of RSA and other asymmetric algorithms depends on the difficulty of factoring large numbers. Factoring large numbers is the strength of Shor's algorithm. Many public key cryptographies use prime factorization to generate keys, but Shor's algorithm could, in theory, break asymmetric cryptography with quantum computers, given their ability to conduct complex calculations quickly. Quantum computers could carry out decryption without knowing the private key, according to the Technology and Privacy Unit of the European Data Protection Supervisor. Traditional cryptography faces several challenges that have led to the exploration of more advanced cryptographic techniques
Here are some challenges associated with traditional cryptography:
- The challenge in crypto key management lies in securely generating, storing, distributing, rotating, and revoking keys while addressing compliance, integration, and human error concerns.
- Crypto key distribution requires ensuring the secure and efficient exchange of cryptographic keys among authorized parties, while safeguarding against interception or tampering
- Side channel attacks exploit unintended information leakage during cryptographic operations, potentially compromising their security.
- Traditional cryptographic algorithms, especially those based on factoring large numbers or discrete logarithms are vulnerable to attacks by quantum computers.
- Certificate management, revocation and trust in the certificate authorities(CAs) can be challenging due to complexity & cost.
- Traditional cryptographic systems may face scalaibility issues, particularly when it comes to large-scale systems or networks
Unlocking Quantum Cryptography: Advantages and Benefits
Quantum cryptography, with its ability to provide secure communication channels using the principles of quantum mechanics, holds immense potential for revolutionizing various aspects of our daily lives. Quantum cryptography offers several advantages over traditional cryptography. First, it provides provable security, meaning that the security of the communication is guaranteed by the laws of physics, not by the computational complexity or the secrecy of the algorithms. Second, it enables long-distance and high-speed communication, as qubits can be transmitted through optical fibers or free space with minimal loss or interference. Third, it allows for new applications, such as quantum digital signatures, quantum secret sharing, and quantum voting, that can enhance the functionality and trustworthiness of cryptographic protocols. While still in the early stages of development and deployment, there are several real-world applications where quantum cryptography is being explored and implemented.
Real-World Applications of Quantum Cryptography:
- Financial Services: Implementing quantum-resistant encryption methods, such as quantum key distribution, financial institutions can safeguard sensitive data, secure transactions, and protect against emerging threats.
- Data Centers and Cloud Computing: By using quantum-resistant encryption algorithms and implementing QKD to establish secure channels between data centers and users, organizations can ensure the confidentiality and integrity of their data in cloud environments.
- Internet of Things (IoT) Security: With quantum-resistant algorithms and secure communication channels, the integrity and confidentiality of IoT data can be preserved, mitigating potential risks.
- Defense and National Security: Quantum cryptography can enhance their capabilities by enabling the secure transmission of classified information, protecting military communications, and ensuring the integrity of critical infrastructure.
- Healthcare and Medical Data: Quantum cryptography ensures security for protecting electronic health records, ensuring patient privacy, and preventing unauthorized access or tampering of medical data.
- Authentication and Identity Management: Quantum-resistant cryptographic algorithms can be employed to secure digital identities, prevent identity theft, and ensure the integrity of user authentication processes.
- Secure Elections and Voting Systems: Quantum cryptography boosts electoral security through tamper-proof encryption and secure channels, protecting vote confidentiality and election integrity.
Quantum-secure Algorithms
Quantum-secure algorithms, also known as post-quantum algorithms, are cryptographic algorithms designed to resist attacks by quantum computers. They are being developed in response to the potential threat posed by quantum computers, which could break many of the currently deployed cryptographic schemes, such as RSA and ECC, through algorithms like Shor's algorithm.
Here are some types of post-quantum algorithms:
- Lattice-based cryptography relies on the hardness of certain problems related to lattices, such as the Shortest Vector Problem (SVP) or the Learning With Errors (LWE) problem. Examples include the NTRUEncrypt encryption scheme and the NTRUSign digital signature scheme.
- Code-based cryptography is based on the hardness of certain problems related to error-correcting codes, such as the decoding problem. Examples include the McEliece cryptosystem and the BIKE key encapsulation mechanism.
- Hash-based cryptography relies on cryptographic hash functions for digital signatures and other cryptographic tasks. Examples include the Merkle signature scheme and the XMSS digital signature scheme.
- Multivariate polynomial-based cryptography is based on the hardness of solving systems of multivariate polynomial equations. Examples include the Unbalanced Oil and Vinegar (UOV) signature scheme and the Rainbow signature scheme.
- Isogeny-based cryptography relies on the difficulty of computing isogenies between elliptic curves. Examples include the Supersingular Isogeny Diffie-Hellman (SIDH) key exchange protocol.
- Supersingular Isogeny-based cryptography is a specific type of isogeny-based cryptography that exploits certain properties of supersingular elliptic curves.
- Lattice-based signature schemes: Apart from encryption schemes, lattice-based cryptography also offers signature schemes that are resistant to quantum attacks. Examples include the BLISS and Dilithium signature schemes.
These are just some of the major categories of post-quantum cryptographic algorithms. Each category encompasses various specific schemes, each with its own strengths, weaknesses, and mathematical underpinnings.
Conclusion:
In conclusion, the necessity of quantum cryptography over traditional cryptography is underscored by the imminent threat posed by quantum computers to widely used cryptographic algorithms. As quantum computing technology advances, the vulnerability of traditional cryptographic systems becomes increasingly apparent. Quantum cryptography provides a paradigm shift by leveraging the principles of quantum mechanics to create inherently secure communication channels. The deployment of quantum-resistant algorithms, quantum key distribution (QKD), and other quantum cryptographic techniques is crucial for ensuring the long-term security of sensitive information in the face of evolving technological landscapes. As organizations recognize the importance of preparing for a quantum-safe future, the adoption of quantum cryptography emerges as a strategic imperative, offering unparalleled security assurances in the era of quantum computing.
Microland is poised to assist organizations in devising a strategic roadmap for system transitions following the release of the new post-quantum cryptographic standard. Leveraging its expertise in managing expansive compute estates and staying abreast of the swift advancements in quantum computing.
As the field of post-quantum cryptography continues to evolve, new algorithms and techniques are being developed and evaluated for their security and efficiency. Quantum cryptography, while promising in its security properties, also has several limitations:
- Physical Implementation Challenges: Quantum cryptography protocols often require specialized hardware for their implementation, These components can be expensive to manufacture and operate, limiting the scalability of quantum cryptography systems.
- Limited Communication Range: Practical implementations of Quantum key distribution (QKD) are typically limited to relatively short distances, such as a few hundred kilometers through optical fibers.
- Key Exchange Rate: The key exchange rate in QKD protocols is lower than classical methods due to factors like the probabilistic nature of single-photon sources and the need for error correction and privacy amplification, which consume extra bandwidth and reduce the effective key generation rate.
- Security Assumptions: Quantum cryptography protocols rely on certain physical assumptions, such as the absence of loopholes in the underlying principles of quantum mechanics. While these assumptions are well-supported by experimental evidence, they are not immune to potential future discoveries or advances in quantum technology that could undermine their validity.
- Vulnerabilities to Side-channel Attacks: Practical quantum cryptography systems may face vulnerability from side-channel attacks, potentially compromising security by targeting classical communication channels for key reconciliation and error correction, independent of exploiting quantum properties.
- Cost and Complexity: Quantum cryptography systems can be costly to deploy and maintain, requiring specialized equipment and expertise for their operation. The complexity of these systems can also pose challenges for integration with existing infrastructure and protocols.
- Interoperability: Interoperability challenges among quantum cryptography protocols hinder secure communication between systems using varied standards or technologies; ongoing standardization efforts aim to alleviate this issue, though achieving broad compatibility remains challenging.
Despite these limitations, quantum cryptography offers unique security advantages, particularly in scenarios where strong security guarantees are paramount, such as in government, defense, and financial applications. Ongoing research and development efforts aim to overcome these limitations and unlock the full potential of quantum cryptography for practical deployment in real-world scenarios.
NIST(National Institute of Standards and Technology ) partnering with DHS(Department of Homeland Security) has created the timeline as shown below:
Source: https://www.dhs.gov/publication/preparing-post-quantum-cryptography-infographic